Small Is Beautiful, Part 2: Testing, Testing
Published: 18 February 2020
The Southern Great Plains is a prime location to evaluate new ground instruments and networks
This is the second article in a series about small campaigns held in 2019 at ARM’s Southern Great Plains atmospheric observatory.
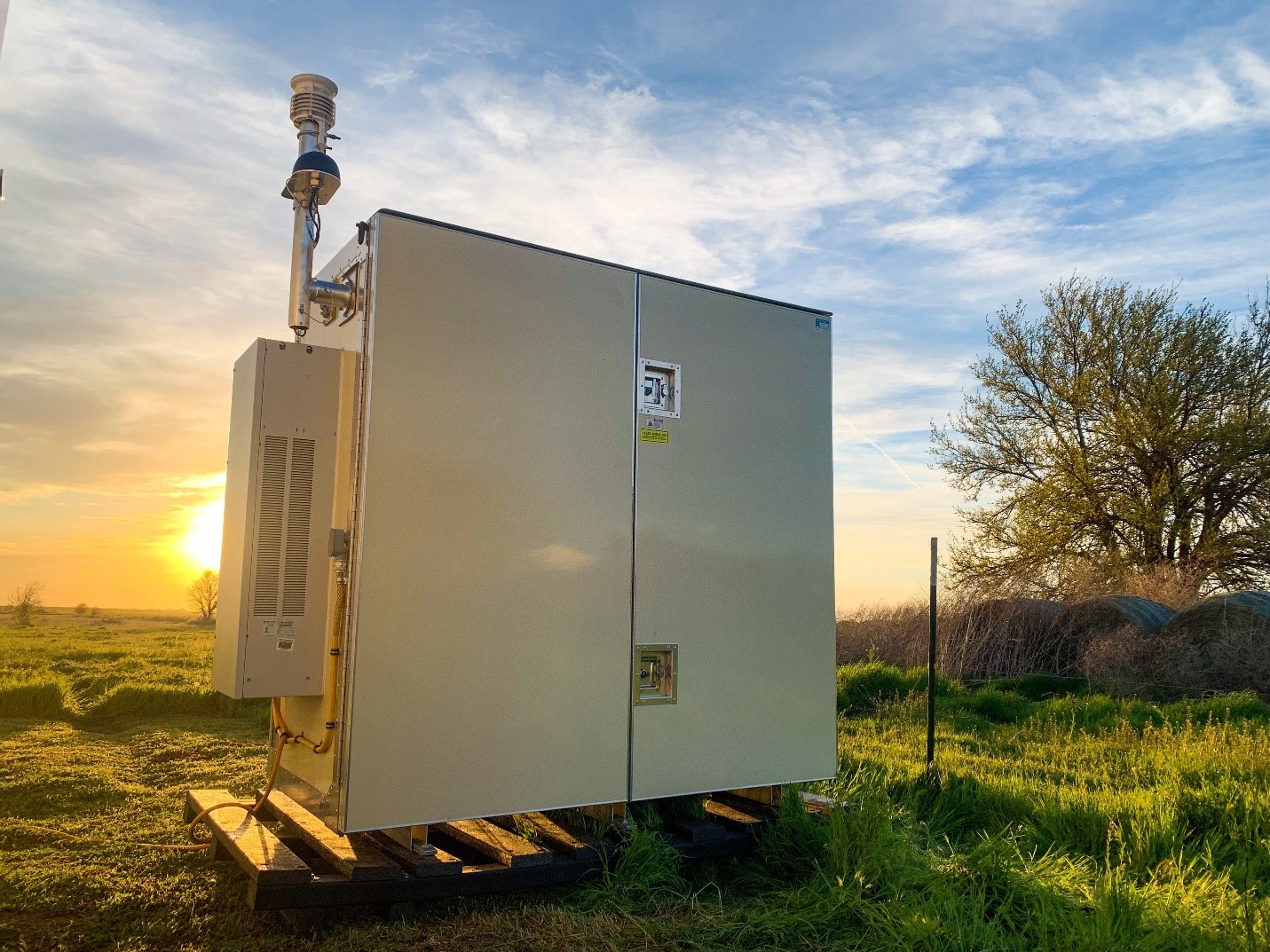
Small campaigns employing tethered balloon systems took to the skies in 2019 at the Southern Great Plains (SGP) atmospheric observatory, managed by the U.S. Department of Energy’s Atmospheric Radiation Measurement (ARM) user facility. But there was also plenty of experimental action involving ground instruments.
ARM defines a small campaign as one costing $300,000 or less. Small campaigns also often deploy guest instruments and involve fewer researchers, instruments, and time than large-scale campaigns.
One small ground campaign took place at the SGP in the spring and summer of 2019. Tammy Weckwerth, an instrument expert from the National Center for Atmospheric Research’s Earth Observing Laboratory in Boulder, Colorado, led a group of scientists and engineers testing a network of five differential absorption lidars (DIALs).
DIALs profile water vapor in the absence of precipitation, which are difficult measurements to take in great detail.
Traditional ways to measure water vapor fall short, says Weckwerth. Surface instruments measure from just one point on the ground. Radiosondes, launched only periodically, collect bytes of data many kilometers apart from other radiosondes.
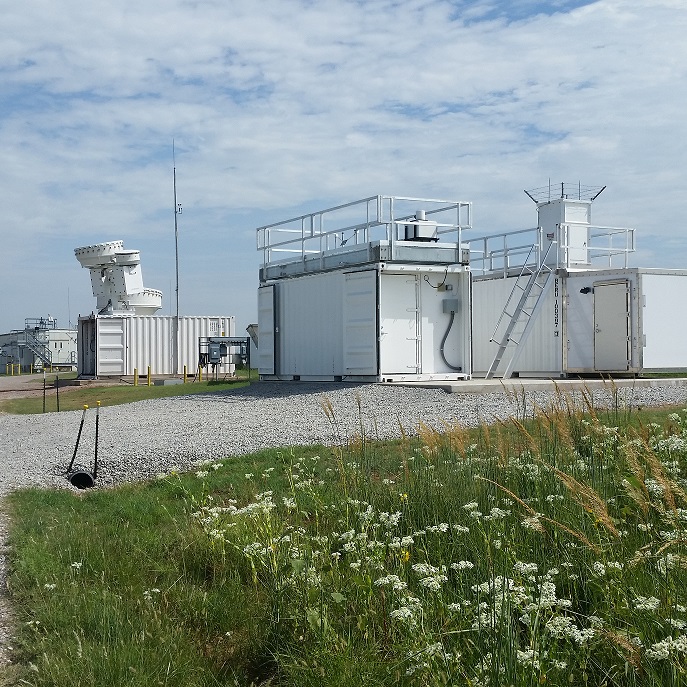
Meanwhile, existing advanced lidar systems, such as the ARM Raman lidar deployed at the SGP, can measure water vapor profiles, but they are typically expensive, one-of-a-kind research instruments.
Lower-cost remote-sensing alternatives would fill a significant gap in observing systems. They could be deployed in networks to provide information on water vapor vertical profiles across larger spatial areas.
Weckwerth’s experiment was part of a joint development project with Montana State University funded by the National Science Foundation and intended to build a network of five micropulse DIALS (MPDs).
The MPD Network Demonstration Project at the SGP was the first attempt to demonstrate the operational efficacy of an unattended MPD network that collects continuous vertical profiles of atmospheric water vapor during the spring-summer convective storm season.
The SGP-tested network is designed to improve and supplement current data-gathering techniques.
Water vapor profiles are one of the key atmospheric thermodynamic variables needed to understand convective storms. (Temperature is another.) Yet such profiles are under-observed, says Weckwerth.
Continuous water vapor data also provide insight into how thunderstorms form and grow, and they are used to improve the predictive accuracy of severe-storm forecasts.
Weckwerth’s team uncovered a glitch during the MPD network test. Humid Midwest summer air built up moisture inside the metal housings of the MPD units. It condensed on interior optics, affecting―though not destroying―the collected data.
Back in Boulder, the containers are undergoing engineering fixes.
Chasing Water Vapor in Clouds
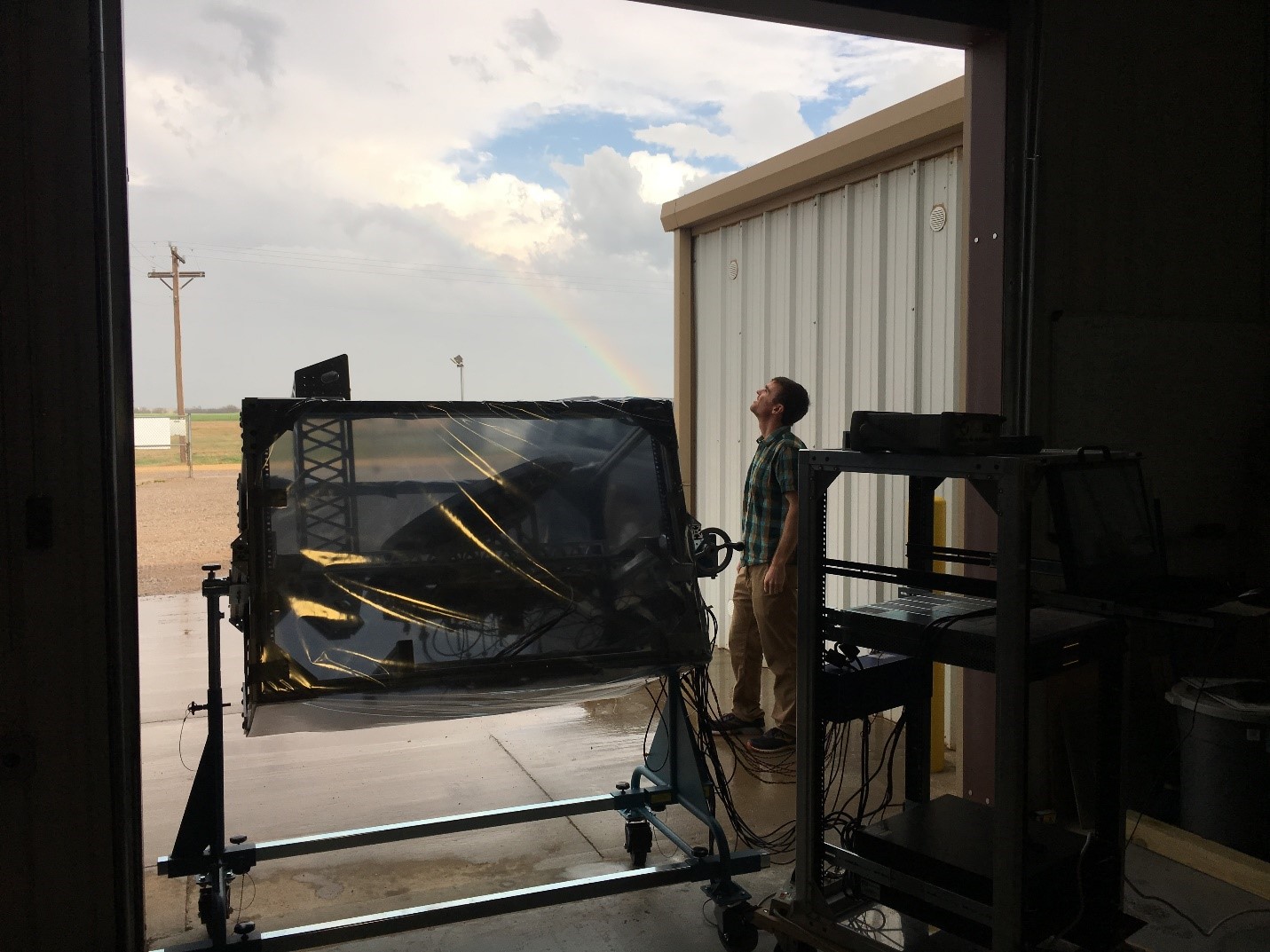
Another small campaign at the SGP focused on water vapor. In a NASA-funded experiment, Matthew Lebsock of the Jet Propulsion Laboratory (JPL) in Pasadena, California, tried to improve knowledge about water vapor in the atmosphere by focusing on water vapor data inside clouds.
Water vapor condenses and forms clouds when it is saturated. In fast-moving updrafts, the water vapor becomes supersaturated, which boosts the intensity of thunderstorms. Models poorly represent this supersaturation.
There are very few good observations of water vapor within clouds, says Lebsock.
The lidar methods discussed in the profile of Weckwerth’s small campaign do not work within clouds. (The visible lidar signal is quickly attenuated by cloud droplets.) For this reason, Lebsock and his colleagues turned to radar systems that operate at longer wavelengths.
To improve model representations of water vapor, Lebsock led a project at the SGP demonstrating a new differential absorption radar designed to measure humidity profiles inside boundary layer clouds, which form in the lowest two kilometers of the atmosphere, where severe weather originates.
The campaign took place over two weeks in April 2019. An ARM field campaign report appeared in November.
Lebsock and his colleagues at the SGP validated their results by comparing the new radar’s measurements with data from radiosondes. These instrumented balloons are one traditional means of gathering data from inside clouds.
But radiosonde data are limited in space and time to a single profile per balloon launch. The new radar, as described in a 2018 paper in the journal Atmospheric Measurement Techniques, can retrieve humidity profiles from within clouds with an impressive resolution of 200 square meters (about 2,150 square feet). The radar can also take data from as far as 10 kilometers (6.2 miles) away.
The radiosonde-radar data came within 10 percent of matching, says Lebsock―“a huge success.”
It helped that springtime weather at the SGP was stormy and that the SGP has a variety of other instruments for measuring water vapor―a boon for comparing data across instruments.
Next, says Lebsock, the new JPL water vapor radar will join a suite of instruments in a forthcoming NASA push to investigate the thermodynamics of the boundary layer.
The Small, the Distributed, the Inexpensive
“We’re going for a new paradigm. We’re doing this on the cheap.”
Allison McComiskey, lead scientist for the POPSnet-SGP field campaign, which involves printed optical particle spectrometers
By mid-November, only one small campaign remained in place out of those that started at the SGP in 2019: the Pilot Aerosol Microphysics Network for Targeting Climate Model Uncertainty.
The campaign is also called POPSnet-SGP, a reference to its core instrument, the printed optical particle spectrometer (POPS). The compact device is designed to capture data on aerosol number concentrations and size distributions.
To save on cost, manufacturing complexity, and weight, the high-sensitivity POPS instruments are 3D-printed on polymer. They weigh in at a svelte 800 grams (about 28 ounces).
Why a POPS network?
Researchers hypothesize that a spatially dense instrument network will deliver copious data of reasonable quality and could help overcome current aerosol measurement gaps.
The POPSnet-SGP network targets a major source of uncertainty in global earth system models: the representation error of aerosol concentrations. That means data from just one site do not represent the full reality of aerosol concentrations within a model’s large grid box.
Ordinarily, “we make very comprehensive measurements at a single point,” and often with very sophisticated and expensive instruments, says POPSnet-SGP lead scientist Allison McComiskey, an aerosols researcher at Brookhaven National Laboratory in New York.
But with a network of less expensive instruments densely distributed over a wide area, she says, “we’re going for a new paradigm. We’re doing this on the cheap.”
In part, that new paradigm is possible because of a new generation of low-cost, miniaturized instruments originally developed for unmanned aerial systems.
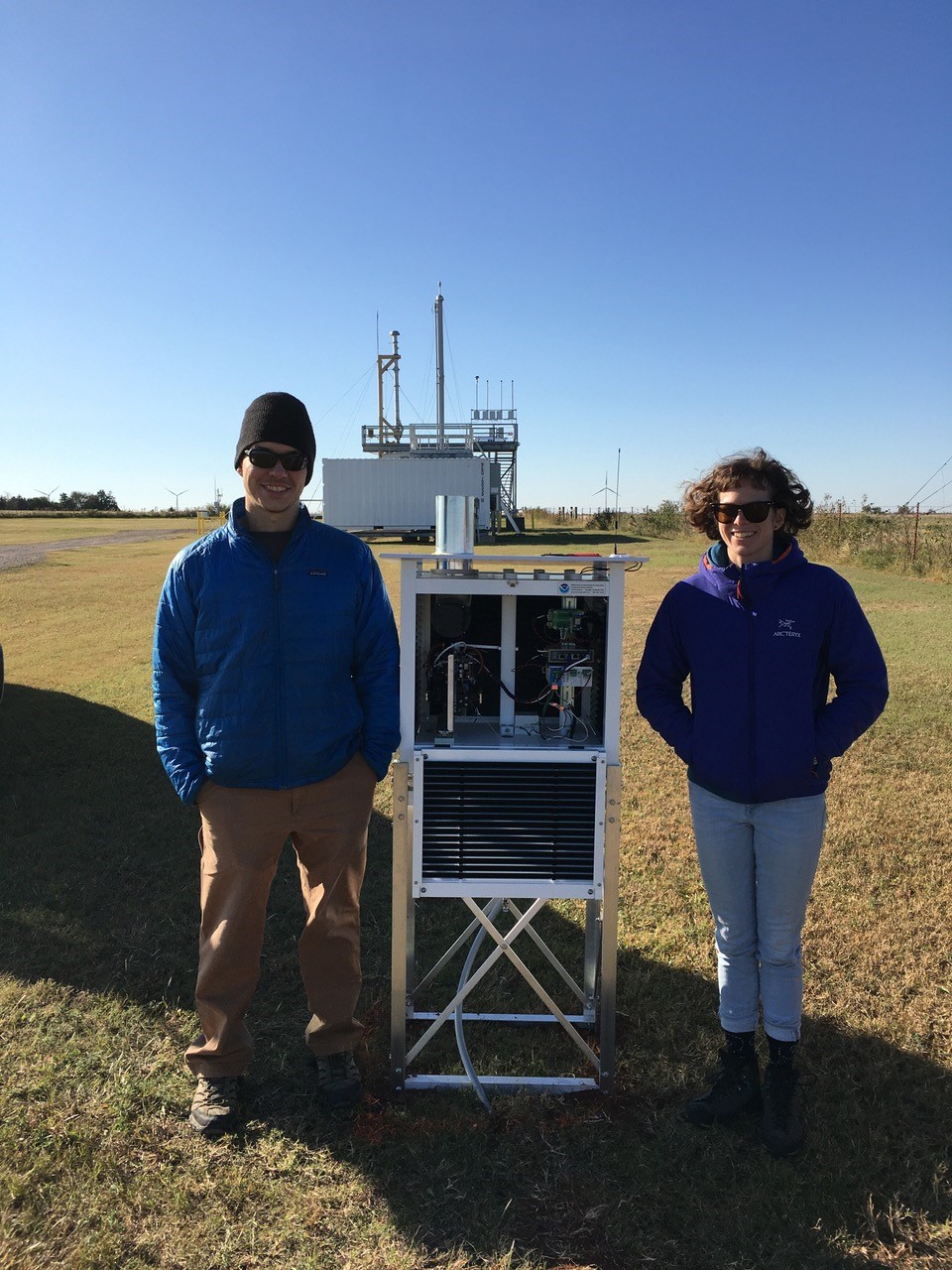
Despite years of research, estimates of aerosol microphysical properties remain uncertain. That means global models are still not reliably accurate.
Meanwhile, there are few networks for measuring aerosol number concentrations, size distributions, and other aerosol microphysical properties. In remote locations, networks for making long-term measurements do not exist at all.
By design, the POPS network will be spread out over a deployment grid at the SGP that is 150 kilometers (93 miles) to each side―the same size as a typical model grid box.
Eventually, the network will consist of 13 sites across the SGP. At each one there will be a POPS and other aerosol instruments designed to operate unattended and housed in metal containers about the size of a mailbox.
During Phase 1 of the installation, in mid-October 2019, technicians deployed 14 instrument packages at seven sites within the SGP: one at the Central Facility near Lamont, Oklahoma, and at six extended facilities. These small, remote small-instrument sites throughout Oklahoma and Kansas radiate outward from the SGP’s heavily instrumented Central Facility.
A Phase 2 deployment of instruments for the pilot aerosol network is scheduled for spring 2020 at other extended facilities. The instruments, which deliver data via cellular connections, are expected to remain in place at the SGP through September 2021.
Joining McComiskey in the POPSnet-SGP campaign are researchers from the Cooperative Institute for Research in Environmental Sciences, the National Oceanic and Atmospheric Administration’s Chemical Sciences Division, Yale University, and the University of Leeds in the United Kingdom.
Keep up with the Atmospheric Observer
Updates on ARM news, events, and opportunities delivered to your inbox
ARM User Profile
ARM welcomes users from all institutions and nations. A free ARM user account is needed to access ARM data.